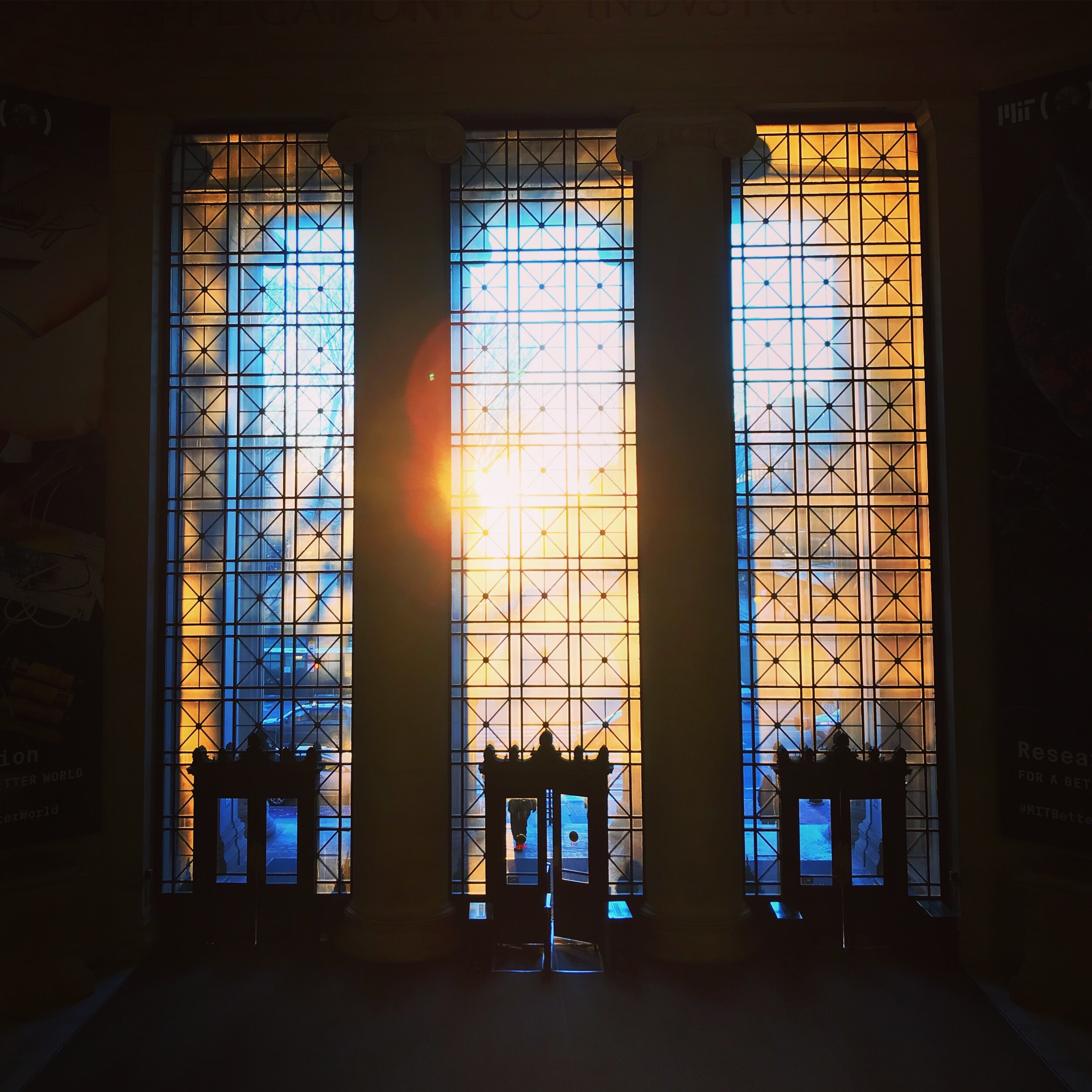
research
Study unlocks nanoscale secrets for designing next-generation solar cells
MIT News, 2024
Study reveals new ways for exotic quasiparticles to “relax”
Next-Generation Photovoltaics
I study the photophysics and optoelectronics of emerging solar cell active layer materials, from synthesis to device fabrication to optical characterization. I am particularly interested in the relationship between optical properties and device performance, and identifying ways in which to close the gap between the theoretical maximum performance and experimentally measured power conversion efficiencies.
Microcavity Exciton-Polaritons
Using 2D perovskites, J-aggregates, and QDs, I study the coupling of confined microcavity photons and photo-generated excitons to form quasi-particles that have characteristics of both light and matter: exciton-polaritons. Exciton-polariton condensates have desirable properties for low-threshold lasing and room-temperature optical computing.
Strongly-Coupled LEDs
Often, exciton-polaritons are generated via optical excitation. For the realization of ubiquitous polaritonic devices, electrically-injected exciton-polaritons are necessary. Toward this end, I study the electrical injection of exciton-polaritons by creating LED structures with imbedded mirrors to serve as a microcavity confining photons from the radiative recombination of active layer excitons.
Exciton-Polaritons
Uncovering Temperature-Dependent Exciton-Polariton Relaxation Mechanisms in Perovskites.
State-of-the-art hybrid perovskites have demonstrated excellent functionality in photovoltaics and light-emitting applications and have emerged as a promising material candidate for exciton- polariton (polariton) optoelectronics. In the strong light-matter coupling regime, polariton formation and Bose-Einstein condensation (BEC) have been demonstrated at room temperature in several perovskite formulations. Thermodynamically, low-threshold BEC requires efficient scattering to the polariton energy dispersion minimum at k|| = 0, and many applications demand precise control of polariton interactions. Thus far, the primary mechanisms by which polaritons relax in perovskites remains unclear. In this work, we perform temperature-dependent measurements of polaritons in low-dimensional hybrid perovskite microcavities and demonstrate high light-matter coupling strengths with a Rabi splitting of ħΩ = 260 ± 5 meV. By embedding the perovskite active layer near the optical field antinode of a wedged microcavity, we are able to tune the Hopfield coefficients by moving the optical excitation along the wedge length and thus decouple the primary polariton relaxation mechanisms in this material for the first time. We observe the thermal activation of a bottleneck regime, and reveal that this effect can be overcome by harnessing intrinsic scattering mechanisms arising from the interplay between the different excitonic species, such as biexciton-assisted polariton relaxation pathways, and isoenergetic intracavity pumping. We demonstrate the dependence of the bottleneck suppression on cavity detuning, and are able to achieve efficient relaxation to k|| = 0 even at cryogenic temperatures. This new understanding contributes to the design of ultra-low-threshold BEC and condensate control by engineering polariton dispersions concomitant with efficient relaxation pathways, leveraging intrinsic material scattering mechanisms for next-generation polariton optoelectronics.
Nature Communications
Photovoltaics
Reduced Recombination.
The ability to reduce energy loss at semiconductor surfaces through passivation or surface field engineering is an essential step in the manufacturing of efficient photovoltaic (PV) and optoelectronic devices. Similarly, surface modification of emerging halide perovskites with quasi-two-dimensional (2D) heterostructures is now ubiquitous to achieve PV power conversion efficiencies (PCEs) >25%, yet a fundamental understanding to how these treatments function is still generally lacking. Here we use a unique combination of depth-sensitive nanoscale characterization techniques to uncover a tunable passivation strategy and mechanism found in perovskite PV devices that were the first to reach the >25% PCE milestone. Namely, treatment with hexylammonium bromide leads to the simultaneous formation of an iodide-rich 2D layer along with a Br halide gradient that extends from defective surfaces and grain boundaries into the bulk three-dimensional (3D) layer. This interface can be optimized to extend the charge carrier lifetime to record values >30 μs and to reduce interfacial recombination velocities to values as low as <7 cm s−1.
Nature Energy
Photon Recycling.
Photon recycling is required for a solar cell to achieve an open-circuit voltage and power conversion efficiency (PCE) approaching the Shockley-Queisser theoretical limit. The achievable performance gains from photon recycling in metal halide perovskite solar cells remain uncertain due to high variability in material quality and the nonradiative recombination rate. We quantify the enhancement due to photon recycling for state-of-the-art triple-cation perovskite films and corresponding solar cells. We show that, at the maximum power point (MPP), the absolute PCE can increase up to 2.0% in the radiative limit, primarily due to a 77 mV increase in the voltage at MPP. For this photoactive layer, even with finite nonradiative recombination, benefits from photon recycling can be achieved when nonradiative lifetimes and external light-emitting diode electroluminescence efficiencies exceed 2 us and 10%, respectively. This analysis quantifies the significance of photon recycling in boosting the real-world performance of perovskite solar cells toward theoretical limits.
Phys Rev Applied, Editors’ Suggestion
External Radiative Efficiency.
Despite rapid advancements in power conversion efficiency in the last decade, perovskite solar cells still perform below their thermodynamic efficiency limits. Non-radiative recombination, in particular, has limited the external radiative efficiency and open circuit voltage in the highest performing devices. We review the historical progress in enhancing perovskite external radiative efficiency and determine key strategies for reaching high optoelectronic quality. Specifically, we focus on non-radiative recombination within the perovskite layer and highlight novel approaches to reduce energy losses at interfaces and through parasitic absorption. By strategically targeting defects, it is likely that the next set of record-performing devices with ultra-low voltage losses will be achieved.
Pure and Appl Chem
Carrier Diffusion.
Energy carrier transport and recombination in emerging semiconductors can be directly monitored with optical microscopy, leading to the measurement of the diffusion coefficient (D), a critical property for the design of efficient optoelectronic devices. The diffusion coefficient is often determine by fitting a time-resolved expanding carrier profile after optical excitation using a Mean Squared Displacement Model. Although this approach has gained widespread adoption, there is no clear consensus as to which material systems, and under what experimental conditions (i.e. laser fluence), it can be applied. Here, we simulate diffusion processes in both excitonic and free carrier semiconductors and present a tailorable MSD Model that can accurately predict D for various materials. For perovskites, it is most accurate when sample D values are > 1 cm2 s−1 and excitation densities are < 1e16 cm−3. At higher carrier densities, non-linear recombination terms can dominate the expansion of the carrier profile and lead to fitting error. We find that differences in grain size and boundary behavior, present in most thin films, can lead to distinct profiles that are not captured by MSD Models. Finally, we present clear strategies to investigate and control energy transport in disordered materials for more effective design and optimization of electronic and optoelectronic devices.
ACS Photonics
LEDs
Strongly-Coupled LEDs.
There are long-standing efforts to achieve electrically-induced lasing in organics, and electrically-driven polariton lasers possess lower thresholds due to the mechanism of lasing in which stimulated scattering processes form a non-equilibrium condensate at k = 0 in momentum space. Designing strongly-coupled LEDs by incorporating a thin reflective layer to serve as a bottom mirror to the top electrode mirror allows for the formation of a cavity mode with which to couple the electrically-pumped exciton. Low-dimensional hybrid organic/inorganic perovskites have been shown to function well as a standard LED active layer material, with the optimal quasi-2D structure of n = 2, 3, or 4 depending on the composition.
We have shown that n = 1 2D perovskites sustain stable, room-temperature exciton-polaritons with large Rabi splittings (~240 meV). By studying 2D materials within high Q-factor microcavity LEDs, we will be able to uniquely measure the response of the material under an electric field with a resolution of several meV. Polaritons are extremely sensitive to electric fields, and we can probe the magnitude of the Stark effect by quantifying small changes in the reflectivity spectrum across the junction. In this way, we can directly measure the Stark effect and polarizability of the exciton. By using a dielectric Bragg reflector instead of a semitransparent Ag mirror, we can achieve high cavity Q-factors, resulting in narrow cavity FWHMs of only ~2-5 meV. The energy of the narrow cavity resonance can be monitored under an applied field to determine with high precision the effect on the active layer material. It is expected that this work could open up the field of exciton-polaritons, which has primarily been driven by applied physicists, to the broader materials and chemistry communities. These studies have far-reaching implications in conducting new reactions using polariton chemistry and in realizing entirely new platforms for efficient lighting, sensing, and energy conversion.
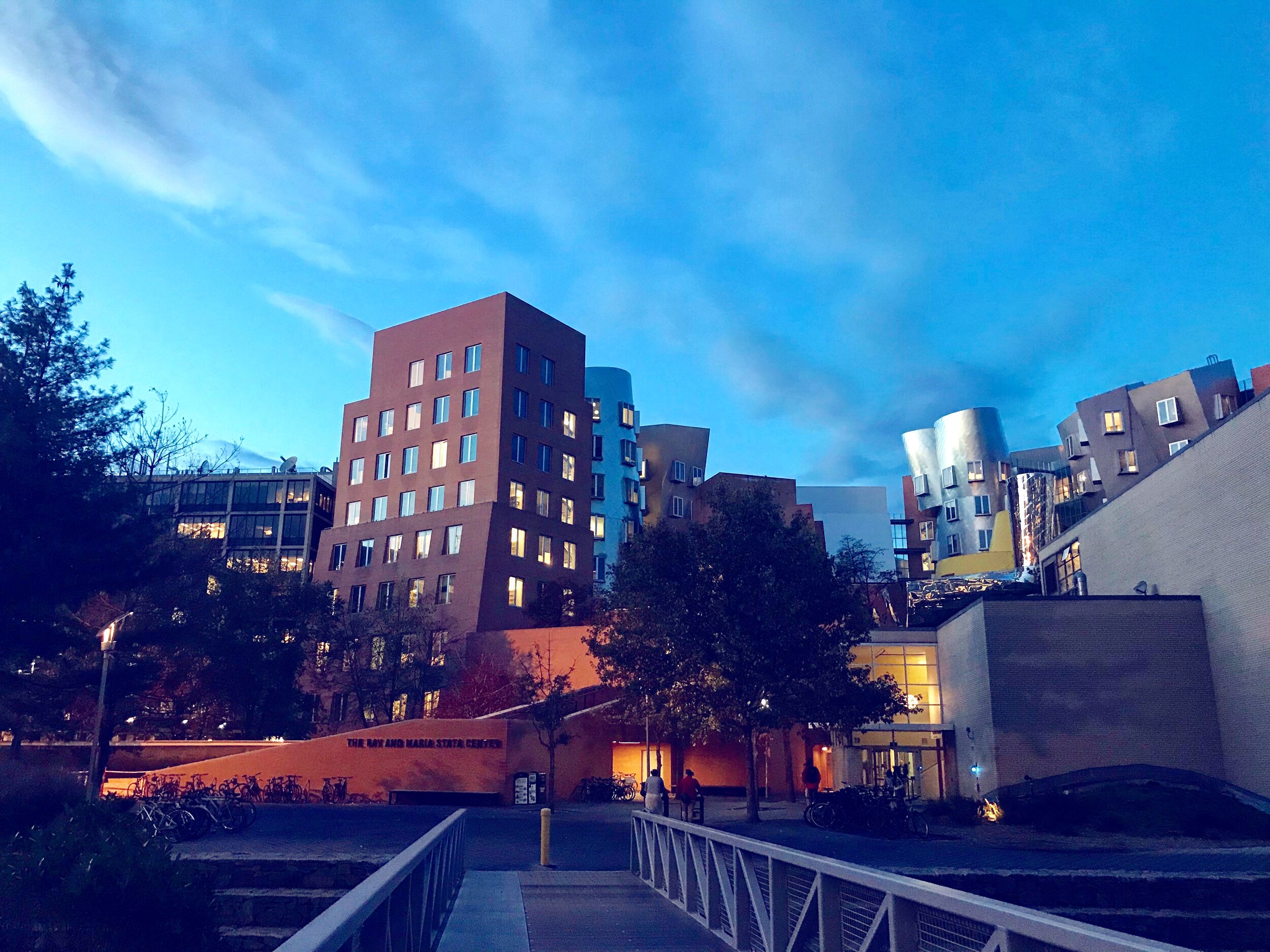
Get in Touch.
Reach out with questions